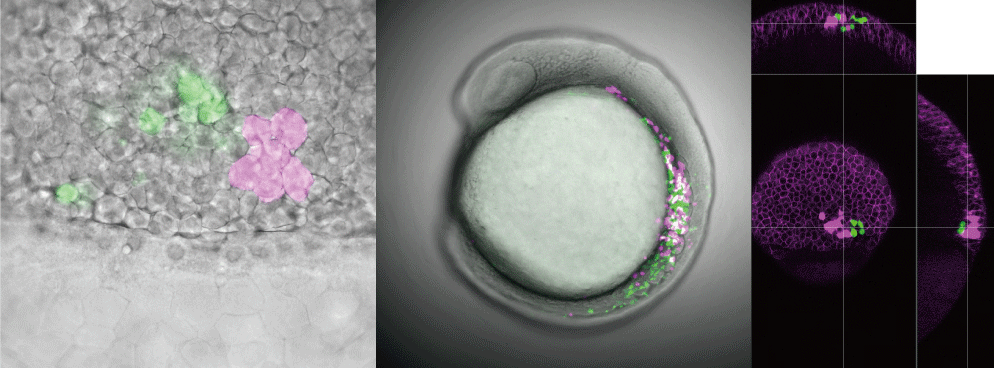
Evolution and development of embryogenesis
All creatures living in the earth have evolved through modification of their embryonic development. How have they achieved this? I study it.
My motivation for science is to understand how embryonic development is achieved, and how such embryogenesis is modified through the course of evolution. To address these, I approach the evolution and development of embryogenesis from various kinds of layers such as gene/genome composition, cell behavior, tissue architecture, organ morphogenesis and body homeostasis. Followings are the current projects I am focusing on.
Evolution and development of the heart
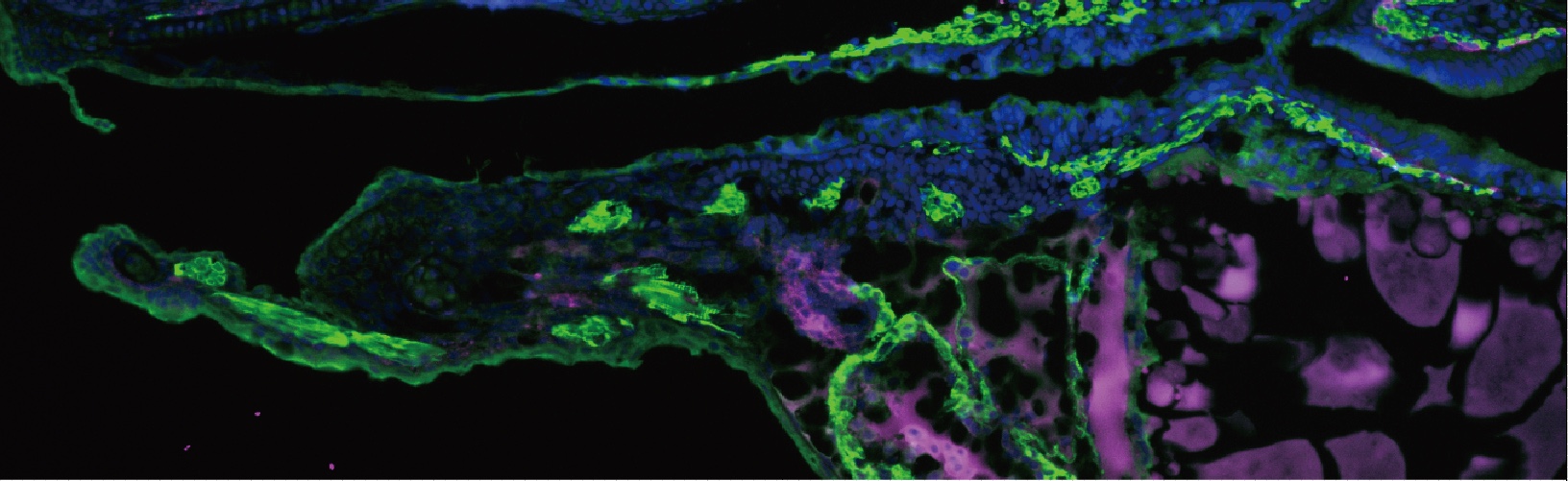
The heart is the first organ to develop and function during embryonic development, and by this mean sometimes it is regarded as a "life" itself. The morphology of the heart is tightly related to its function, and we can see the great diversity of its form depending on habitats or environments (Figure 1). The question I address is; how such morphological diversity has emerged? I study embryonic development of the heart, and by comparing its form among different species I approach the evolution and development of the heart.
From recent publication
Vertebrate heart is composed by four chambers; from caudal to rostral, sinus venosus, ventricle, atrium and outflow tract. Tetrapod has sophisticated their circulatory system by dividing atrium and ventricle into left and right chambers each through forming septum, which leads to separation of arterial and venous blood. In contrast to this, teleost has taken another approach; transformation of their outflow tract. Outflow tract in non-teleost vertebrates is composed by mainly cardiac muscle like other heart components, however, outflow tract in teleost is composed by mainly smooth muscle and has unique characters, and is called "bulbus arteriosus (BA)" (Figure 2). The BA has an important role of acting as a 'wind-kessel' function, which absorbs the energy of a bolus of blood ejected by the ventricle by achieving elastic expansion and recoil, and smooths the pressure wave down the arterial tree. The BA is also unique in its abundance of elastin, which is considered to have a central role for its wind-kessel function. From these characters, BA has been regarded as a one of the most important evolutionary novelties in teleost. However, the mechanism underlying development of BA had been largely unknown; how did/do teleost, in their evolution and development, convert cell types of the BA from cardiac into smooth muscle cells and accumulate specialized elastic fibers?
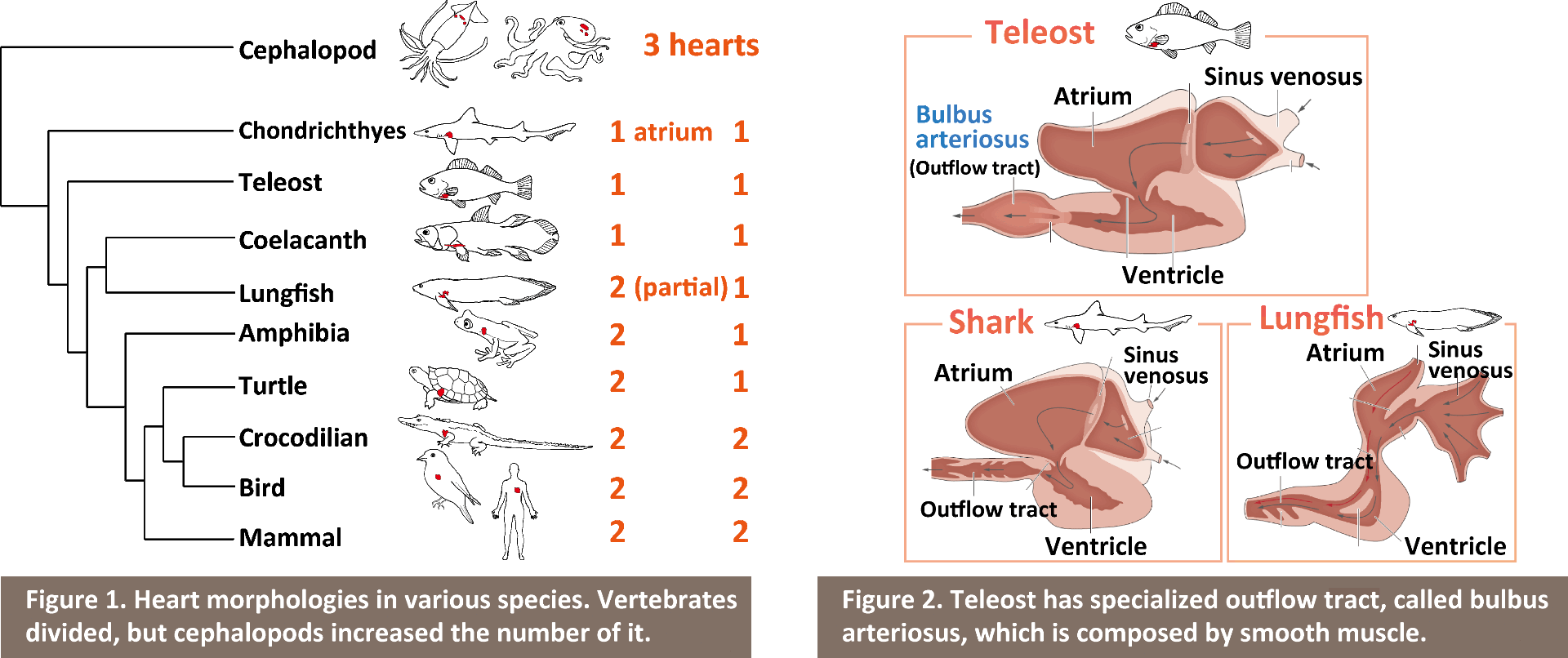
Recently I have discovered that
sub/neo-functionalization of an elastin
gene endows teleost with evolutionary novelty BA. elastin is a gene which encodes extracellular matrix (ECM) and it
was duplicated by teleost-specific whole genome duplication, resulting in two
parallogues, elastin a and elastin b. One of them, elastin b, is expressed only in outflow
tract and converts cardiac precursor cells into smooth muscle. When elastin b is compromised, cardiac muscle
cells are ectopically formed in BA without alteration of cardiac precursor
cells. This suggests that elastin b
regulates cell fate determination of BA cells through mechanotransduction.
Indeed, recently it has been shown that ECMs can change cell fate determination
depending their own stiffness. In summary, this study illustrates a new
mechanism for generating evolutionary novelty; alternation of the extracellular
environment by an ECM alters cell determination through mechanotransduction,
which generates a new organ in evolution and development.
References:
Moriyama et al., Nature Communications. 2016
Moriyama and Koshiba. Briefings in Functional Genomics. 2018
Katano and Moriyama et al., Development Growth and Differentiation. 2019
Cell behavior, mechanics and the evolution of gastrulation
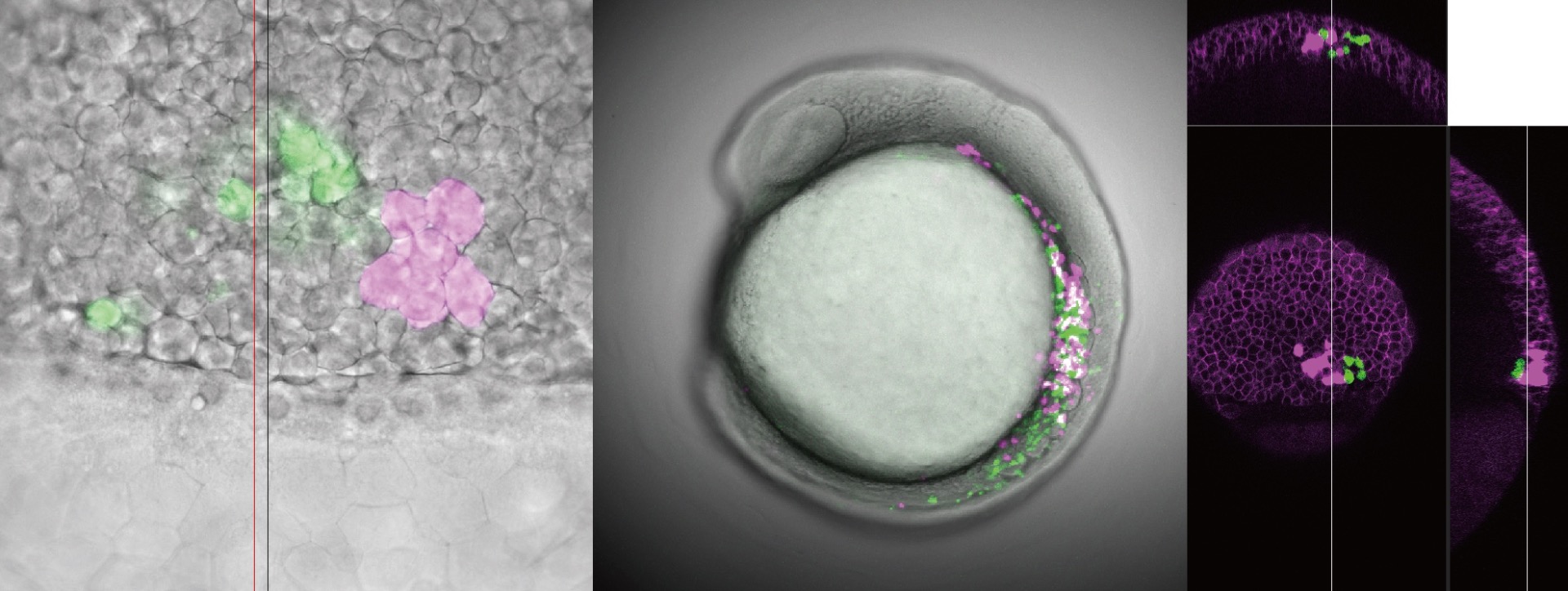
Gastrulation is the process establishing three germ layer, ectoderm, endoderm and mesoderm, in early embryogenesis in all bilaterians. The process of gastrulation is quite diversed during the evolution (Figure 3). However, we can see the universal aspect for the evolution of gastrulation; cells migrate inward and specified their fate into endoderm, mesoderm and ectoderm in order from the inside of the embryo. Gastrulation alters their mode with keeping its own quintessence. I study cell behavior, mechanics and biophysical properties during gastrulation utilizing live-imaging techniques for zebrafish embryos. Then, by comparing the unveiling mechanisms with different species, I approach the developmental constraint for the evolution of gastrulation.
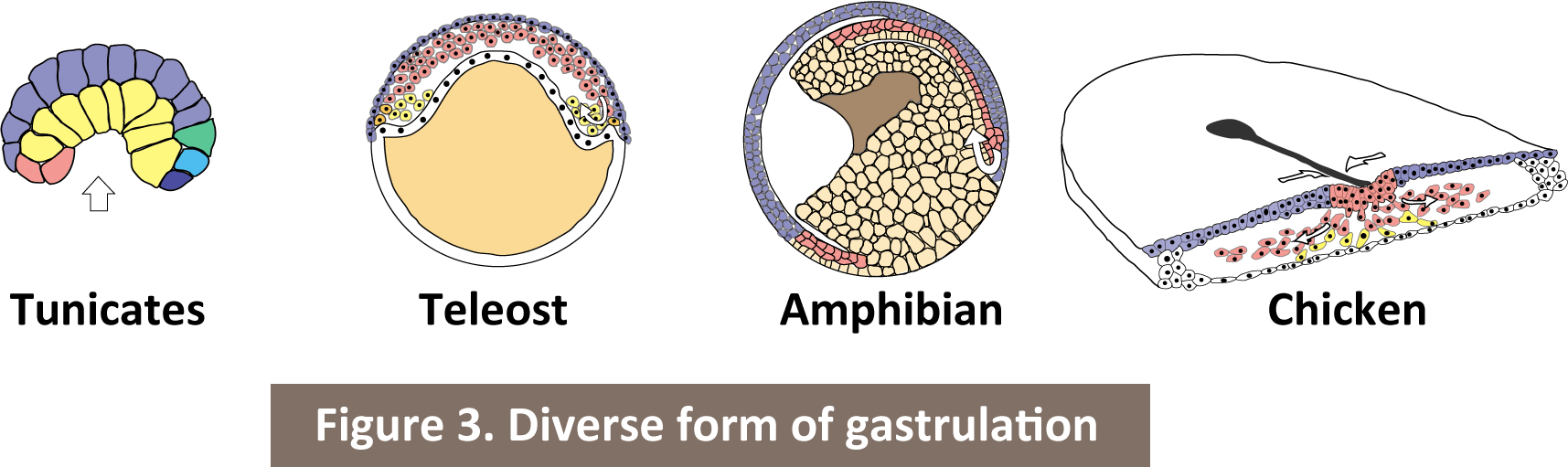
Gene duplication as a means of morphological/functional evolution
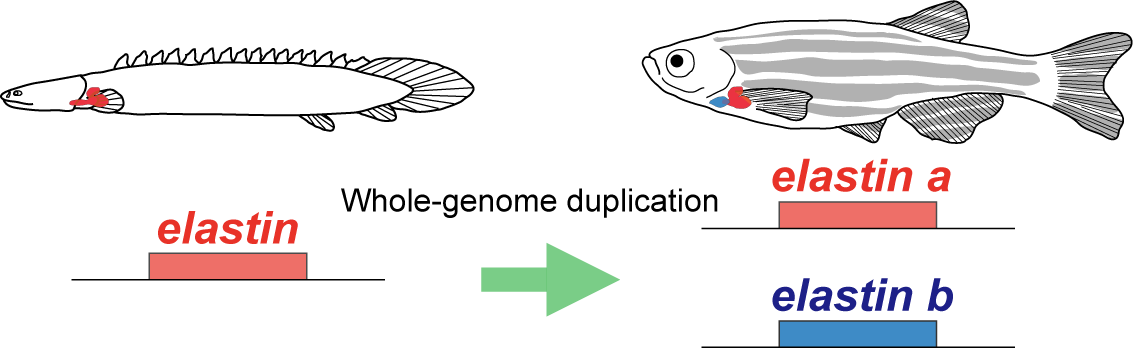
Gene and/or whole-genome duplication are phenomena that contribute to supplying genetic row materials for morphological/function evolution, which leads to adaptations for species' living environments. Susumu Ohno put forward the hypothesis that genome duplication and subsequent sub/neo-functionalization are a potent force generating morphological/functional evolution (Ohno. Evolution by Gene Duplication. 1970), and it has been supported a series of studies thereafter. So, what kind of genes are linked to specific morphological/functional evolution? In many cases, one of the duplicated genes becomes pseudogene (loss of function) and it makes difficult to address this point.
Through the study of heart evolution as described above, I found that elastin gene duplication by teleost-specific 3rd round whole-genome duplication (3R WGD) and subsequent sub/neo-functionalization endows teleost with elastic BA. Riding on the way of the study, I am seeking for the duplicated genes generating unique characters seen in teleost, by focusing on 3rd WGD.
References:
Ohno. "Evolution by Gene Duplication" Springer-Verlag. 1970
Acquisition of dorsal identity and the evolution of caudal skeleton
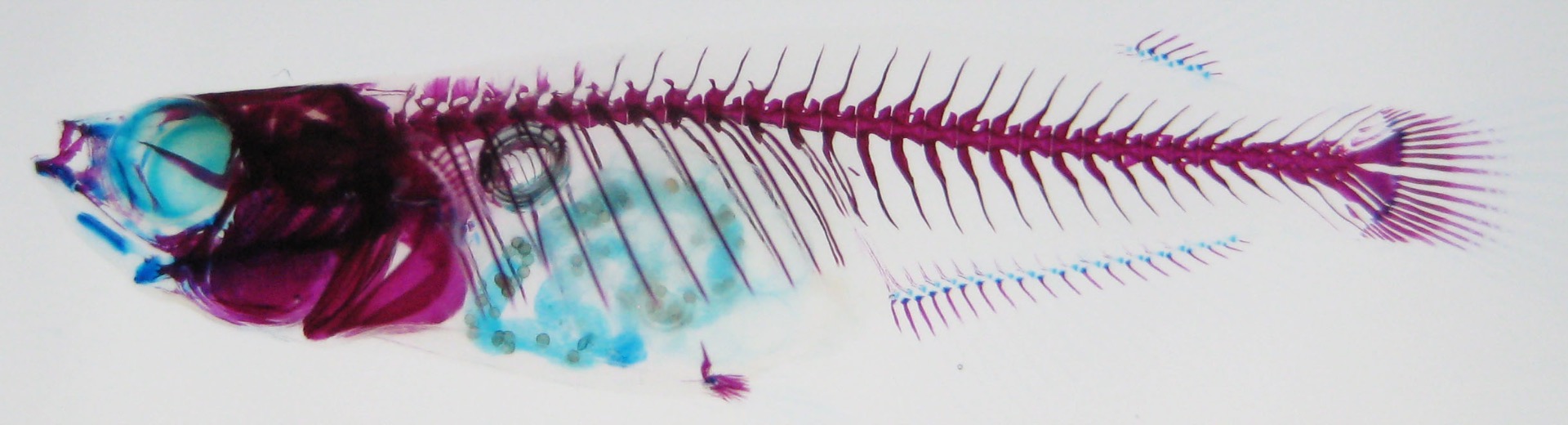
Like the case of heart evolution, other body parts have also changed their morphology for adapting living environments. Teleosts are the most successful group in vertebrates and represent 95% of all living fish species, by adapting aquatic environment. This explosive radiation is achieved partly through acquisition of specialized caudal-fin skeleton. In teleost, caudal fin is superficially symmetric, but the caudal part of vertebral column tilts strongly dorsally, quite asymmetric internally. This teleost caudal fin is called "homocercal fin" and is regarded as one of the most important evolutionary novelties in teleost. However, how homocercal fin is generated in embryo and acquired during evolution had been largely unknown. I revealed the molecular mechanism underlying the morphogenesis of homocercal fin utilizing medaka spontaneous mutant Double anal fin (Da) that loses dorsoventral asymmetry in trunk and tail region. I found that Da is an enhancer mutant for zic1/zic4 and loses the expression of these genes in somites and tail by insertion of the huge transposon, while the expression in neural tube is unaffected. During the embryogenesis, somites are formed through the trunk and tail, but after that caudal-most somites are fused and generates mesenchyme that is abundant with sclerotome, resulting in emergence of unique structure. I named it as "caudal-skeleton forming mesenchyme: CSM". Taken together, I found that zic1/zic4 are co-opted to dorsal CSM during vertebrate evolution and it endows teleost with asymmetric homocercal fin.
References:
Moriyama et al., Current Biology. 2012
Morphological evolution of paired appendages
Limbs (arms/legs) in tetrapods are homologous to the paired fins (pectral/pelvic fins) in fish respectively. In the course of vertebrate evolution, these paired fins transformed into limbs and its phenomena is called as 'fin to limb transition'. I focus on the similarity and differences of the developmental mechanisms between fins and limbs, and want to understand the developmental constraints for it.
References:
Moriyama et al., Zoological Science. 2019
Kawahata et al., Scientific Reports. 2019
Okamoto and Moriyama et al., Development, Growth and Differentiation. 2019